Optical sensors are used in applications including cameras, lidar, autonomous navigation, free-space optical communications, smartphones, scientific imaging, and defense. In each of these applications, the sensor’s sensitivity, bandwidth, and dynamic range are key to overall performance, determining factors such as a system’s maximum range, resolution, and data transfer speed.
This invention allows imaging a dynamic scene over an extreme range of timescales simultaneously - seconds to picoseconds - and doing so passively, without much light, and without any timing signals from the light source(s) emitting it. The system applies a computational algorithm to the output stream of photon timestamps produced by the detector, recovering ultra-fast variations in the intensity of incident light. Specifically, the invention makes it possible to reconstruct a pixel’s time-varying flux (i.e. light intensity) from a discrete, asynchronous, stochastic, and never-ending stream of photon arrival times, showing for the first time, that:
- Flux reconstruction is possible even if it varies orders of magnitude faster than the timespan between consecutive photon arrivals, and
- They can compute its Fourier decomposition directly from the inkling photon stream.
Using it in combination with a free-running SPAD (single-photon avalanche diode) camera, the inventors demonstrate never-before-seen abilities, such as:
- Observing light in flight passively,
- Intensity imaging at GHz speeds, and
- Passively recording video that captures variations at everyday timescales – but can be slowed down by a factor of a billion to show the propagation of light itself.
BENEFITS
The main advantages of this technology are:
- Passive and asynchronous. Compared to existing optical sensors, the approach achieves temporal resolution on the order of picoseconds without requiring synchronization to an active source, enabling passive observation of faint, ultra-fast optical signals that are not synchronized to the detector.
- Leverages existing single-photon detection technology. The system is compatible with existing single-photon detector technologies, including CMOS SPADs, which are highly scalable and have already been deployed in consumer electronics (e.g., the Apple iPhone).
- Opens up new applications. The system enables detection of extremely weak and faint sources; for example, it could be used for detection and localization of lidar systems, self-localization from ambient pulsed light sources, or high-bandwidth optical communications.
Current optical sensors either (1) require synchronization to a controlled light source to measure ultra-fast signals, or (2) they are limited by noise and so do not reach single-photon sensitivity. Optical sensing is especially challenging when photons arrive at the sensor at a rate that is slower than the signals being measured. This occurs in time-of-flight sensing, for example, where picosecond or nanosecond-scale signals must be measured from a handful of photons arriving in millisecond timespans. It also applies to setting where a computer vision system must operate reliably in severely light-starved environments (e.g. near total darkness). To overcome this issue, existing solutions rely on synchronized sensing—they pair a sensor with an ultra-fast (e.g., picosecond) strobe light, plus extra hardware to precisely synchronize the sensor with the light. By capturing the same event under strobed illumination repeatedly—often millions of times—enough photons are collected to recover an ultra-fast signal of interest. However, this paradigm is very limiting and cumbersome, and does not allow for asynchronous, passive measurements —for example, detection of ambient lidar signals, measurement of multiple asynchronous laser sources, or characterization of chaotic broadband optical signals. It is also exclusively limited to measuring ultrafast phenomena, as synchronized sensing cannot measure slowly-varying signals.
While fast photodiodes and avalanche photodiodes offer passive, asynchronous sensing at bandwidths exceeding 10 GHz, they do not reach single photon sensitivity and may fail to detect weak signals at ultra-fast timescales. Thus, there are no existing solutions for ultra-broadband optical sensing with single-photon sensitivity.
APPLICATIONS
The team has demonstrated the technology for the following applications:
- Simultaneous detection and characterization of ultra-fast (picosecond) laser signals from multiple lidar systems.
- Monitoring and detection of ultra-broadband optical signals (Hz to 10+ GHz) over durations from seconds to tens of minutes.
Sector End-use Applications:
-
High-speed photonics and sensing. Ultra-broadband capture of faint optical signals in photonics applications that require extreme bandwidth and sensitivity (exceeding that of conventional fast photodetectors or avalanche photodiodes).
-
Lidar. The technology has potential utility for long-range or fast-scanning lidar systems. It may also enable bi-static lidar systems that operate asynchronously (i.e., without requiring an explicit connection between the transmitter and receiver).
-
Security/Defense/Surveillance. Detection of faint optical signals or fast-moving objects in low light is crucial for security, defense, and surveillance applications. Passive ultra-broadband single-photon sensing is uniquely positioned to have advantages in this sector.
-
A new generation of ultra-flexible video cameras. Conventional 2D cameras can passively record video at rates that are limited by the exposure time of individual video frames. The most advanced video cameras based on SPAD technologies are limited to approximately 100K binary frames per second; other technologies that enable ultra-fast readout are limited to about 1 million frames per second, require extreme amounts of light to acquire video with reasonable levels of noise. This invention enables acquisition of continuous video at controllable rates from 30 frames per second (or less) to over 200 billion frames per second. Moreover, acquisition can take place using already available camera hardware technologies (free-running SPAD pixels) and conventional light levels.
-
Sync-less imaging with multiple, ultra-fast lasers. numerous imaging systems, from automotive Lidars and consumer depth cameras to fluorescence lifetime microscopes (FLIM), rely on accurate synchronization of a laser with one or more light detectors. Such synchronization requires considerable hardware infrastructure and high-speed electronics to achieve it. This approach makes it possible for these techniques to be employed without any sync whatsoever, simplifying the hardware needs as well as the flexibility of the underlying imaging systems.
STATUS
-
PCT filed Feb 2024
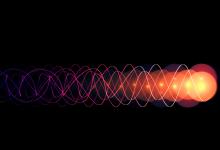